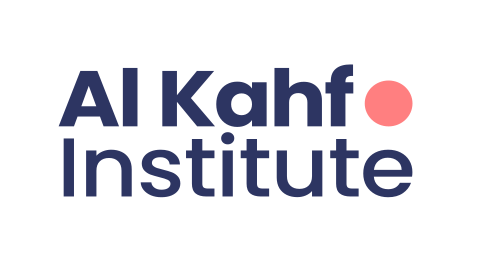
Sun
The Sun is the star at the centre of the Solar System. It is a massive, nearly perfect sphere of hot plasma, heated to incandescence by nuclear fusion reactions in its core, radiating the energy from its surface mainly as visible light and infrared radiation with 10% at ultraviolet energies. It is by far the most important source of energy for life on Earth. The Sun has been an object of veneration in many cultures. It has been a central subject for astronomical research since antiquity.
The Sun orbits the Galactic Center at a distance of 24,000 to 28,000 light-years. Its distance from Earth defines the astronomical unit, which is about 1.496×108 kilometres or about 8 light-minutes. Its diameter is about 1,391,400 km (864,600 mi), 109 times that of Earth. The Sun's mass is about 330,000 times that of Earth, making up about 99.86% of the total mass of the Solar System. Roughly three-quarters of the Sun's mass consists of hydrogen (~73%); the rest is mostly helium (~25%), with much smaller quantities of heavier elements, including oxygen, carbon, neon, and iron.
The Sun is a G-type main-sequence star (G2V), informally called a yellow dwarf, though its light is actually white. It formed approximately 4.6 billion[a] years ago from the gravitational collapse of matter within a region of a large molecular cloud. Most of this matter gathered in the centre; the rest flattened into an orbiting disk that became the Solar System. The central mass became so hot and dense that it eventually initiated nuclear fusion in its core. Every second, the Sun's core fuses about 600 billion kilograms (kg) of hydrogen into helium and converts 4 billion kg of matter into energy.
About 4 to 7 billion years from now, when hydrogen fusion in the Sun's core diminishes to the point where the Sun is no longer in hydrostatic equilibrium, its core will undergo a marked increase in density and temperature which will cause its outer layers to expand, eventually transforming the Sun into a red giant. After the red giant phase, models suggest the Sun will shed its outer layers and become a dense type of cooling star (a white dwarf), and no longer produce energy by fusion, but will still glow and give off heat from its previous fusion for perhaps trillions of years. After that, it is theorised to become a super dense black dwarf, giving off negligible energy.
Etymology
The English word sun developed from Old English sunne. Cognates appear in other Germanic languages, including West Frisian sinne, Dutch zon, Low German Sünn, Standard German Sonne, Bavarian Sunna, Old Norse sunna, and Gothic sunnō. All these words stem from Proto-Germanic *sunnōn.[17][18] This is ultimately related to the word for sun in other branches of the Indo-European language family, though in most cases a nominative stem with an l is found, rather than the genitive stem in n, as for example in Latin sōl, ancient Greek ἥλιος (hēlios), Welsh haul and Czech slunce, as well as (with *l > r) Sanskrit स्वर् (svár) and Persian خور (xvar). Indeed, the l-stem survived in Proto-Germanic as well, as *sōwelan, which gave rise to Gothic sauil (alongside sunnō) and Old Norse prosaic sól (alongside poetic sunna), and through it the words for sun in the modern Scandinavian languages: Swedish and Danish sol, Icelandic sól, etc.[18]
The principal adjectives for the Sun in English are sunny for sunlight and, in technical contexts, solar (/ˈsoʊlər/),[3] from Latin sol.[19] From the Greek helios comes the rare adjective heliac (/ˈhiːliæk/).[20] In English, the Greek and Latin words occur in poetry as personifications of the Sun, Helios (/ˈhiːliəs/) and Sol (/ˈsɒl/),[2][1] while in science fiction Sol may be used to distinguish the Sun from other stars. The term sol with a lowercase s is used by planetary astronomers for the duration of a solar day on another planet such as Mars.[21]
The astronomical symbol for the Sun is a circle with a central dot, .[22] It is used for such units as M☉ (Solar mass), R☉ (Solar radius) and L☉ (Solar luminosity).[23][24] The scientific study of the Sun is called heliology.[25]
General characteristics
Size comparison of the Sun, all eight planets of the Solar System, other bodies, and some larger stars. The Sun is 1.4 million kilometres (4.643 light-seconds) wide, about 109 times wider than Earth, or four times the Lunar distance, and contains 99.86% of all Solar System mass.
The Sun is a G-type main-sequence star that makes up about 99.86% of the mass of the Solar System.[26] It has an absolute magnitude of +4.83, estimated to be brighter than about 85% of the stars in the Milky Way, most of which are red dwarfs.[27][28] It is more massive than 95% of the stars within 7 pc (23 ly).[29] The Sun is a Population I, or heavy-element-rich,[b] star.[30] Its formation approximately 4.6 billion years ago may have been triggered by shockwaves from one or more nearby supernovae.[31][32] This is suggested by a high abundance of heavy elements in the Solar System, such as gold and uranium, relative to the abundances of these elements in so-called Population II, heavy-element-poor, stars. The heavy elements could most plausibly have been produced by endothermic nuclear reactions during a supernova, or by transmutation through neutron absorption within a massive second-generation star.[30]
The Sun is by far the brightest object in the Earth's sky, with an apparent magnitude of −26.74.[33][34] This is about 13 billion times brighter than the next brightest star, Sirius, which has an apparent magnitude of −1.46.[35]
One astronomical unit (about 150 million kilometres; 93 million miles) is defined as the mean distance between the centres of the Sun and the Earth. The instantaneous distance varies by about ±2.5 million kilometres (1.6 million miles) as Earth moves from perihelion around 3 January to aphelion around 4 July.[36] At its average distance, light travels from the Sun's horizon to Earth's horizon in about 8 minutes and 20 seconds,[37] while light from the closest points of the Sun and Earth takes about two seconds less. The energy of this sunlight supports almost all life[c] on Earth by photosynthesis,[38] and drives Earth's climate and weather.[39]
The Sun does not have a definite boundary, but its density decreases exponentially with increasing height above the photosphere.[40] For the purpose of measurement, the Sun's radius is considered to be the distance from its centre to the edge of the photosphere, the apparent visible surface of the Sun.[41] The roundness of the Sun is the relative difference between its radius at its equator, Req, and at its pole, Rpol, called the oblateness,[42]Δ⊙=(Req−Rpol)/Rpol.
The value is difficult to measure. Atmospheric distortion means the measurement must be done on satellites; the value is very small meaning very precise technique is needed.[43]
The oblateness was once proposed to be sufficient to explain the perihelion precession of Mercury but Einstein proposed that general relativity could explain the precession using a spherical Sun.[43] When high precision measurements of the oblateness became available via the Solar Dynamics Observatory[44] and the Picard satellite[42] the measured value was even smaller than expected,[43] 8.2 x 10−6, or 8 parts per million. These measurements determined the Sun to be the natural object closest to a perfect sphere ever observed..[45] The oblateness value remains constant independent of solar irradiation changes.[42] The tidal effect of the planets is weak and does not significantly affect the shape of the Sun.[46]
Rotation
Main article: Solar rotation
The Sun rotates faster at its equator than at its poles. This differential rotation is caused by convective motion due to heat transport and the Coriolis force due to the Sun's rotation. In a frame of reference defined by the stars, the rotational period is approximately 25.6 days at the equator and 33.5 days at the poles. Viewed from Earth as it orbits the Sun, the apparent rotational period of the Sun at its equator is about 28 days.[47] Viewed from a vantage point above its north pole, the Sun rotates counterclockwise around its axis of spin.[d][48]
A survey of solar analogues suggests the early Sun was rotating up to ten times faster than it does today. This would have made the surface much more active, with greater X-ray and UV emission. Sun spots would have covered 5–30% of the surface.[49] The rotation rate was gradually slowed by magnetic braking, as the Sun's magnetic field interacted with the outflowing solar wind.[50] A vestige of this rapid primordial rotation still survives at the Sun's core, which rotates at a rate of once per week; four times the mean surface rotation rate.[51][52]
Composition
See also: Molecules in stars
The Sun consists mainly of the elements hydrogen and helium. At this time in the Sun's life, they account for 74.9% and 23.8%, respectively, of the mass of the Sun in the photosphere.[53] All heavier elements, called metals in astronomy, account for less than 2% of the mass, with oxygen (roughly 1% of the Sun's mass), carbon (0.3%), neon (0.2%), and iron (0.2%) being the most abundant.[54]
The Sun's original chemical composition was inherited from the interstellar medium out of which it formed. Originally it would have been about 71.1% hydrogen, 27.4% helium, and 1.5% heavier elements.[53] The hydrogen and most of the helium in the Sun would have been produced by Big Bang nucleosynthesis in the first 20 minutes of the universe, and the heavier elements were produced by previous generations of stars before the Sun was formed, and spread into the interstellar medium during the final stages of stellar life and by events such as supernovae.[55]
Since the Sun formed, the main fusion process has involved fusing hydrogen into helium. Over the past 4.6 billion years, the amount of helium and its location within the Sun has gradually changed. The proportion of helium within the core has increased from about 24% to about 60% due to fusion, and some of the helium and heavy elements have settled from the photosphere toward the centre of the Sun because of gravity. The proportions of heavier elements are unchanged. Heat is transferred outward from the Sun's core by radiation rather than by convection (see Radiative zone below), so the fusion products are not lifted outward by heat; they remain in the core,[56] and gradually an inner core of helium has begun to form that cannot be fused because presently the Sun's core is not hot or dense enough to fuse helium. In the current photosphere, the helium fraction is reduced, and the metallicity is only 84% of what it was in the protostellar phase (before nuclear fusion in the core started). In the future, helium will continue to accumulate in the core, and in about 5 billion years this gradual build-up will eventually cause the Sun to exit the main sequence and become a red giant.[57]
The chemical composition of the photosphere is normally considered representative of the composition of the primordial Solar System.[58] Typically, the solar heavy-element abundances described above are measured both by using spectroscopy of the Sun's photosphere and by measuring abundances in meteorites that have never been heated to melting temperatures. These meteorites are thought to retain the composition of the protostellar Sun and are thus not affected by the settling of heavy elements. The two methods generally agree well.[59]
Core
Main article: Solar core
The core of the Sun extends from the centre to about 20–25% of the solar radius.[60] It has a density of up to 150 g/cm3[61][62] (about 150 times the density of water) and a temperature of close to 15.7 million kelvin (K).[62] By contrast, the Sun's surface temperature is about 5800 K. Recent analysis of SOHO mission data favours the idea that the core is rotating faster than the radiative zone outside it.[60] Through most of the Sun's life, energy has been produced by nuclear fusion in the core region through the proton–proton chain; this process converts hydrogen into helium.[63] Currently, 0.8% of the energy generated in the Sun comes from another sequence of fusion reactions called the CNO cycle; the proportion coming from the CNO cycle is expected to increase as the Sun becomes older and more luminous.[64][65]
The core is the only region of the Sun that produces an appreciable amount of thermal energy through fusion; 99% of the Sun's power is generated in the innermost 24% of its radius, and almost no fusion occurs beyond 30% of the radius. The rest of the Sun is heated by this energy as it is transferred outward through many successive layers, finally to the solar photosphere where it escapes into space through radiation (photons) or advection (massive particles).[66][67]
The proton–proton chain occurs around 9.2×1037 times each second in the core, converting about 3.7×1038 protons into alpha particles (helium nuclei) every second (out of a total of ~8.9×1056 free protons in the Sun), or about 6.2×1011 kg/s. However, each proton (on average) takes around 9 billion years to fuse with another using the PP chain.[66] Fusing four free protons (hydrogen nuclei) into a single alpha particle (helium nucleus) releases around 0.7% of the fused mass as energy,[68] so the Sun releases energy at the mass–energy conversion rate of 4.26 billion kg/s (which requires 600 billion kg of hydrogen[69]), for 384.6 yottawatts (3.846×1026 W),[5] or 9.192×1010 megatons of TNT per second. The large power output of the Sun is mainly due to the huge size and density of its core (compared to Earth and objects on Earth), with only a fairly small amount of power being generated per cubic metre. Theoretical models of the Sun's interior indicate a maximum power density, or energy production, of approximately 276.5 watts per cubic metre at the centre of the core,[70] which, according to Karl Kruszelnicki, is about the same power density inside a compost pile.[71]
The fusion rate in the core is in a self-correcting equilibrium: a slightly higher rate of fusion would cause the core to heat up more and expand slightly against the weight of the outer layers, reducing the density and hence the fusion rate and correcting the perturbation; and a slightly lower rate would cause the core to cool and shrink slightly, increasing the density and increasing the fusion rate and again reverting it to its present rate.[72][73]
Rediative Zone
The radiative zone is the thickest layer of the Sun, at 0.45 solar radii. From the core out to about 0.7 solar radii, thermal radiation is the primary means of energy transfer.[74] The temperature drops from approximately 7 million to 2 million kelvins with increasing distance from the core.[62] This temperature gradient is less than the value of the adiabatic lapse rate and hence cannot drive convection, which explains why the transfer of energy through this zone is by radiation instead of thermal convection.[62] Ions of hydrogen and helium emit photons, which travel only a brief distance before being reabsorbed by other ions.[74] The density drops a hundredfold (from 20,000 kg/m3 to 200 kg/m3) between 0.25 solar radii and 0.7 radii, the top of the radiative zone.[74]
Tachocline
Main article: Tachocline
The radiative zone and the convective zone are separated by a transition layer, the tachocline. This is a region where the sharp regime change between the uniform rotation of the radiative zone and the differential rotation of the convection zone results in a large shear between the two—a condition where successive horizontal layers slide past one another.[75] Presently, it is hypothesised that a magnetic dynamo, or solar dynamo, within this layer generates the Sun's magnetic field.[62]
Convective zone
Main article: Convection zone
The Sun's convection zone extends from 0.7 solar radii (500,000 km) to near the surface. In this layer, the solar plasma is not dense or hot enough to transfer the heat energy of the interior outward via radiation. Instead, the density of the plasma is low enough to allow convective currents to develop and move the Sun's energy outward towards its surface. Material heated at the tachocline picks up heat and expands, thereby reducing its density and allowing it to rise. As a result, an orderly motion of the mass develops into thermal cells that carry most of the heat outward to the Sun's photosphere above. Once the material diffusively and radiatively cools just beneath the photospheric surface, its density increases, and it sinks to the base of the convection zone, where it again picks up heat from the top of the radiative zone and the convective cycle continues. At the photosphere, the temperature has dropped 350-fold to 5,700 K (9,800 °F) and the density to only 0.2 g/m3 (about 1/10,000 the density of air at sea level, and 1 millionth that of the inner layer of the convective zone).[62]
The thermal columns of the convection zone form an imprint on the surface of the Sun giving it a granular appearance called the solar granulation at the smallest scale and supergranulation at larger scales. Turbulent convection in this outer part of the solar interior sustains "small-scale" dynamo action over the near-surface volume of the Sun.[62] The Sun's thermal columns are Bénard cells and take the shape of roughly hexagonal prisms.[76]
Photosphere
The visible surface of the Sun, the photosphere, is the layer below which the Sun becomes opaque to visible light.[77] Photons produced in this layer escape the Sun through the transparent solar atmosphere above it and become solar radiation, sunlight. The change in opacity is due to the decreasing amount of H− ions, which absorb visible light easily.[77] Conversely, the visible light perceived is produced as electrons react with hydrogen atoms to produce H− ions.[78][79]
The photosphere is tens to hundreds of kilometres thick, and is slightly less opaque than air on Earth. Because the upper part of the photosphere is cooler than the lower part, an image of the Sun appears brighter in the centre than on the edge or limb of the solar disk, in a phenomenon known as limb darkening.[77] The spectrum of sunlight has approximately the spectrum of a black-body radiating at 5,772 K (9,930 °F),[12] interspersed with atomic absorption lines from the tenuous layers above the photosphere. The photosphere has a particle density of ~1023 m−3 (about 0.37% of the particle number per volume of Earth's atmosphere at sea level). The photosphere is not fully ionised—the extent of ionisation is about 3%, leaving almost all of the hydrogen in atomic form.[80]
References
- "Sol". Oxford English Dictionary (Online ed.). Oxford University Press. (Subscription or participating institution membership required.)
- ^ Jump up to:
- a b "Helios". Lexico UK English Dictionary. Oxford University Press. Archived from the original on 27 March 2020.
- ^ Jump up to:
- a b "solar". Oxford English Dictionary (Online ed.). Oxford University Press. (Subscription or participating institution membership required.)
- ^ Pitjeva, E. V.; Standish, E. M. (2009). "Proposals for the masses of the three largest asteroids, the Moon–Earth mass ratio and the Astronomical Unit". Celestial Mechanics and Dynamical Astronomy. 103 (4): 365–372. Bibcode:2009CeMDA.103..365P. doi:10.1007/s10569-009-9203-8. ISSN 1572-9478. S2CID 121374703. Archived from the original on 9 July 2019. Retrieved 13 July 2019.
- ^ Jump up to:
- a b c d e f g h i j k l m n o p Williams, D. R. (1 July 2013). "Sun Fact Sheet". NASA Goddard Space Flight Center. Archived from the original on 15 July 2010. Retrieved 12 August 2013.
- ^ Zombeck, Martin V. (1990). Handbook of Space Astronomy and Astrophysics 2nd edition. Cambridge University Press. Archived from the original on 3 February 2021. Retrieved 13 January 2016.
- ^ Asplund, M.; Grevesse, N.; Sauval, A. J. (2006). "The new solar abundances – Part I: the observations". Communications in Asteroseismology. 147: 76–79. Bibcode:2006CoAst.147...76A. doi:10.1553/cia147s76. ISSN 1021-2043. S2CID 123824232.
- ^ "Eclipse 99: Frequently Asked Questions". NASA. Archived from the original on 27 May 2010. Retrieved 24 October 2010.
- ^ Francis, Charles; Anderson, Erik (June 2014). "Two estimates of the distance to the Galactic Centre". Monthly Notices of the Royal Astronomical Society. 441 (2): 1105–1114. arXiv:1309.2629. Bibcode:2014MNRAS.441.1105F. doi:10.1093/mnras/stu631. S2CID 119235554.
- ^ Hinshaw, G.; Weiland, J. L.; Hill, R. S.; Odegard, N.; Larson, D.; et al. (2009). "Five-year Wilkinson Microwave Anisotropy Probe observations: data processing, sky maps, and basic results". The Astrophysical Journal Supplement Series. 180 (2): 225–245. arXiv:0803.0732. Bibcode:2009ApJS..180..225H. doi:10.1088/0067-0049/180/2/225. S2CID 3629998.
- ^ Jump up to:
- a b c d e f "Solar System Exploration: Planets: Sun: Facts & Figures". NASA. Archived from the original on 2 January 2008.
- ^ Jump up to:
- a b c Prša, Andrej; Harmanec, Petr; Torres, Guillermo; et al. (1 August 2016). "NOMINAL VALUES FOR SELECTED SOLAR AND PLANETARY QUANTITIES: IAU 2015 RESOLUTION B3 * †". The Astronomical Journal. 152 (2): 41. arXiv:1510.07674. Bibcode:2016AJ....152...41P. doi:10.3847/0004-6256/152/2/41. ISSN 0004-6256.
- ^ Jump up to:
- a b Bonanno, A.; Schlattl, H.; Paternò, L. (2002). "The age of the Sun and the relativistic corrections in the EOS". Astronomy and Astrophysics. 390 (3): 1115–1118. arXiv:astro-ph/0204331. Bibcode:2002A&A...390.1115B. doi:10.1051/0004-6361:20020749. S2CID 119436299.
- ^ Connelly, J. N.; Bizzarro, M.; Krot, A. N.; Nordlund, Å.; Wielandt, D.; Ivanova, M. A. (2 November 2012). "The Absolute Chronology and Thermal Processing of Solids in the Solar Protoplanetary Disk". Science. 338 (6107): 651–655. Bibcode:2012Sci...338..651C. doi:10.1126/science.1226919. PMID 23118187. S2CID 21965292.(registration required)
- ^ Gray, David F. (November 1992). "The Inferred Color Index of the Sun". Publications of the Astronomical Society of the Pacific. 104 (681): 1035–1038. Bibcode:1992PASP..104.1035G. doi:10.1086/133086.
- ^ "The Sun's Vital Statistics". Stanford Solar Center. Archived from the original on 14 October 2012. Retrieved 29 July 2008. Citing Eddy, J. (1979). A New Sun: The Solar Results From Skylab. NASA. p. 37. NASA SP-402. Archived from the original on 30 July 2021. Retrieved 12 July 2017.
- ^ Barnhart, R. K. (1995). The Barnhart Concise Dictionary of Etymology. HarperCollins. p. 776. ISBN 978-0-06-270084-1.
- ^ Jump up to:
- a b Orel, Vladimir (2003). A Handbook of Germanic Etymology. Leiden: Brill. p. 41. ISBN 978-9-00-412875-0 – via Internet Archive.
- ^ Little, William; Fowler, H. W.; Coulson, J. (1955). "Sol". Oxford Universal Dictionary on Historical Principles (3rd ed.). ASIN B000QS3QVQ.
- ^ "heliac". Oxford English Dictionary (Online ed.). Oxford University Press. (Subscription or participating institution membership required.)
- ^ "Opportunity's View, Sol 959 (Vertical)". NASA. 15 November 2006. Archived from the original on 22 October 2012. Retrieved 1 August 2007.
- ^ Allen, Clabon W.; Cox, Arthur N. (2000). Cox, Arthur N. (ed.). Allen's Astrophysical Quantities (4th ed.). Springer. p. 2. ISBN 978-0-38-798746-0.
- ^ "solar mass". Oxford Reference. Retrieved 26 May 2024.
- ^ Weissman, Paul; McFadden, Lucy-Ann; Johnson, Torrence (18 September 1998). Encyclopedia of the Solar System. Academic Press. pp. 349, 820. ISBN 978-0-08-057313-7.
- ^ "heliology". Collins Dictionary. Collins. Retrieved 24 November 2024.
- ^ Woolfson, M. (2000). "The origin and evolution of the solar system" (PDF). Astronomy & Geophysics. 41 (1): 12. Bibcode:2000A&G....41a..12W. doi:10.1046/j.1468-4004.2000.00012.x. Archived (PDF) from the original on 11 July 2020. Retrieved 12 April 2020.
- ^ Than, K. (2006). "Astronomers Had it Wrong: Most Stars are Single". Space.com. Archived from the original on 21 December 2010. Retrieved 1 August 2007.
- ^ Lada, C. J. (2006). "Stellar multiplicity and the initial mass function: Most stars are single". Astrophysical Journal Letters. 640 (1): L63 – L66. arXiv:astro-ph/0601375. Bibcode:2006ApJ...640L..63L. doi:10.1086/503158. S2CID 8400400.
- ^ Robles, José A.; Lineweaver, Charles H.; Grether, Daniel; Flynn, Chris; Egan, Chas A.; Pracy, Michael B.; Holmberg, Johan; Gardner, Esko (September 2008). "A Comprehensive Comparison of the Sun to Other Stars: Searching for Self-Selection Effects". The Astrophysical Journal. 684 (1): 691–706. arXiv:0805.2962. Bibcode:2008ApJ...684..691R. doi:10.1086/589985. hdl:1885/34434. Retrieved 24 May 2024.
- ^ Jump up to:
- a b Zeilik, M. A.; Gregory, S. A. (1998). Introductory Astronomy & Astrophysics (4th ed.). Saunders College Publishing. p. 322. ISBN 978-0-03-006228-5.
- ^ Connelly, James N.; Bizzarro, Martin; Krot, Alexander N.; Nordlund, Åke; Wielandt, Daniel; Ivanova, Marina A. (2 November 2012). "The Absolute Chronology and Thermal Processing of Solids in the Solar Protoplanetary Disk". Science. 338 (6107): 651–655. Bibcode:2012Sci...338..651C. doi:10.1126/science.1226919. PMID 23118187. S2CID 21965292.
- ^ Falk, S. W.; Lattmer, J. M.; Margolis, S. H. (1977). "Are supernovae sources of presolar grains?". Nature. 270 (5639): 700–701. Bibcode:1977Natur.270..700F. doi:10.1038/270700a0. S2CID 4240932.
- ^ Burton, W. B. (1986). "Stellar parameters". Space Science Reviews. 43 (3–4): 244–250. doi:10.1007/BF00190626. S2CID 189796439.
- ^ Bessell, M. S.; Castelli, F.; Plez, B. (1998). "Model atmospheres broad-band colors, bolometric corrections and temperature calibrations for O–M stars". Astronomy and Astrophysics. 333: 231–250. Bibcode:1998A&A...333..231B.
- ^ Hoffleit, D.; et al. (1991). "HR 2491". Bright Star Catalogue (5th Revised ed.). CDS. Bibcode:1991bsc..book.....H.
- ^ "Equinoxes, Solstices, Perihelion, and Aphelion, 2000–2020". US Naval Observatory. 31 January 2008. Archived from the original on 13 October 2007. Retrieved 17 July 2009.
- ^ Cain, Fraser (15 April 2013). "How long does it take sunlight to reach the Earth?". phys.org. Archived from the original on 2 March 2022. Retrieved 2 March 2022.
- ^ "The Sun's Energy: An Essential Part of the Earth System". Center for Science Education. Retrieved 24 May 2024.
- ^ "The Sun's Influence on Climate". Princeton University Press. 23 June 2015. Retrieved 24 May 2024.
- ^ Beer, J.; McCracken, K.; von Steiger, R. (2012). Cosmogenic Radionuclides: Theory and Applications in the Terrestrial and Space Environments. Springer. p. 41. ISBN 978-3-642-14651-0.
- ^ Phillips, K. J. H. (1995). Guide to the Sun. Cambridge University Press. p. 73. ISBN 978-0-521-39788-9.
- ^ Jump up to:
- a b c Meftah, M.; Irbah, A.; Hauchecorne, A.; Corbard, T.; Turck-Chièze, S.; Hochedez, J.-F.; Boumier, P.; Chevalier, A.; Dewitte, S.; Mekaoui, S.; Salabert, D. (March 2015). "On the Determination and Constancy of the Solar Oblateness". Solar Physics. 290 (3): 673–687. Bibcode:2015SoPh..290..673M. doi:10.1007/s11207-015-0655-6. ISSN 0038-0938.
- ^ Jump up to:
- a b c Gough, Douglas (28 September 2012). "How Oblate Is the Sun?". Science. 337 (6102): 1611–1612. Bibcode:2012Sci...337.1611G. doi:10.1126/science.1226988. ISSN 0036-8075. PMID 23019636.
- ^ Kuhn, J. R.; Bush, R.; Emilio, M.; Scholl, I. F. (28 September 2012). "The Precise Solar Shape and Its Variability". Science. 337 (6102): 1638–1640. Bibcode:2012Sci...337.1638K. doi:10.1126/science.1223231. ISSN 0036-8075. PMID 22903522.
- ^ Jones, G. (16 August 2012). "Sun is the most perfect sphere ever observed in nature". The Guardian. Archived from the original on 3 March 2014. Retrieved 19 August 2013.
- ^ Schutz, B. F. (2003). Gravity from the ground up. Cambridge University Press. pp. 98–99. ISBN 978-0-521-45506-0.
- ^ Phillips, K. J. H. (1995). Guide to the Sun. Cambridge University Press. pp. 78–79. ISBN 978-0-521-39788-9.
- ^ "The Anticlockwise Solar System". Australian Space Academy. Archived from the original on 7 August 2020. Retrieved 2 July 2020.
- ^ Guinan, Edward F.; Engle, Scott G. (June 2009). The Sun in time: age, rotation, and magnetic activity of the Sun and solar-type stars and effects on hosted planets. The Ages of Stars, Proceedings of the International Astronomical Union, IAU Symposium. Vol. 258. pp. 395–408. arXiv:0903.4148. Bibcode:2009IAUS..258..395G. doi:10.1017/S1743921309032050.
- ^ Pantolmos, George; Matt, Sean P. (November 2017). "Magnetic Braking of Sun-like and Low-mass Stars: Dependence on Coronal Temperature". The Astrophysical Journal. 849 (2). id. 83. arXiv:1710.01340. Bibcode:2017ApJ...849...83P. doi:10.3847/1538-4357/aa9061.
- ^ Fossat, E.; Boumier, P.; Corbard, T.; Provost, J.; Salabert, D.; Schmider, F. X.; Gabriel, A. H.; Grec, G.; Renaud, C.; Robillot, J. M.; Roca-Cortés, T.; Turck-Chièze, S.; Ulrich, R. K.; Lazrek, M. (August 2017). "Asymptotic g modes: Evidence for a rapid rotation of the solar core". Astronomy & Astrophysics. 604. id. A40. arXiv:1708.00259. Bibcode:2017A&A...604A..40F. doi:10.1051/0004-6361/201730460.
- ^ Darling, Susannah (1 August 2017). "ESA, NASA's SOHO Reveals Rapidly Rotating Solar Core". NASA. Retrieved 31 May 2024.
- ^ Jump up to:
- a b Lodders, Katharina (10 July 2003). "Solar System Abundances and Condensation Temperatures of the Elements" (PDF). The Astrophysical Journal. 591 (2): 1220–1247. Bibcode:2003ApJ...591.1220L. CiteSeerX 10.1.1.666.9351. doi:10.1086/375492. S2CID 42498829. Archived from the original (PDF) on 7 November 2015. Retrieved 1 September 2015.
Lodders, K. (2003). "Abundances and Condensation Temperatures of the Elements" (PDF). Meteoritics & Planetary Science. 38 (suppl): 5272. Bibcode:2003M&PSA..38.5272L. Archived (PDF) from the original on 13 May 2011. Retrieved 3 August 2008. - ^ Hansen, C. J.; Kawaler, S. A.; Trimble, V. (2004). Stellar Interiors: Physical Principles, Structure, and Evolution (2nd ed.). Springer. pp. 19–20. ISBN 978-0-387-20089-7.
- ^ Hansen, C. J.; Kawaler, S. A.; Trimble, V. (2004). Stellar Interiors: Physical Principles, Structure, and Evolution (2nd ed.). Springer. pp. 77–78. ISBN 978-0-387-20089-7.
- ^ Hansen, C. J.; Kawaler, S. A.; Trimble, V. (2004). Stellar Interiors: Physical Principles, Structure, and Evolution (2nd ed.). Springer. § 9.2.3. ISBN 978-0-387-20089-7.
- ^ Iben, Icko Jnr. (November 1965). "Stellar Evolution. II. The Evolution of a 3 M☉ Star from the Main Sequence Through Core Helium Burning". The Astrophysical Journal. 142: 1447. Bibcode:1965ApJ...142.1447I. doi:10.1086/148429.
- ^ Aller, L. H. (1968). "The chemical composition of the Sun and the solar system". Proceedings of the Astronomical Society of Australia. 1 (4): 133. Bibcode:1968PASA....1..133A. doi:10.1017/S1323358000011048. S2CID 119759834.
- ^ Basu, S.; Antia, H. M. (2008). "Helioseismology and Solar Abundances". Physics Reports. 457 (5–6): 217–283. arXiv:0711.4590. Bibcode:2008PhR...457..217B. doi:10.1016/j.physrep.2007.12.002. S2CID 119302796.
- ^ Jump up to:
- a b García, R.; et al. (2007). "Tracking solar gravity modes: the dynamics of the solar core". Science. 316 (5831): 1591–1593. Bibcode:2007Sci...316.1591G. doi:10.1126/science.1140598. PMID 17478682. S2CID 35285705.
- ^ Basu, Sarbani; Chaplin, William J.; Elsworth, Yvonne; New, Roger; Serenelli, Aldo M. (2009). "Fresh insights on the structure of the solar core". The Astrophysical Journal. 699 (2): 1403–1417. arXiv:0905.0651. Bibcode:2009ApJ...699.1403B. doi:10.1088/0004-637X/699/2/1403. S2CID 11044272.
- ^ Jump up to:
- a b c d e f g "NASA/Marshall Solar Physics". Marshall Space Flight Center. 18 January 2007. Archived from the original on 29 March 2019. Retrieved 11 July 2009.
- ^ Broggini, C. (2003). Physics in Collision, Proceedings of the XXIII International Conference: Nuclear Processes at Solar Energy. XXIII Physics in Collisions Conference. Zeuthen, Germany. p. 21. arXiv:astro-ph/0308537. Bibcode:2003phco.conf...21B. Archived from the original on 21 April 2017. Retrieved 12 August 2013.
- ^ Goupil, M. J.; Lebreton, Y.; Marques, J. P.; Samadi, R.; Baudin, F. (2011). "Open issues in probing interiors of solar-like oscillating main sequence stars 1. From the Sun to nearly suns". Journal of Physics: Conference Series. 271 (1): 012031. arXiv:1102.0247. Bibcode:2011JPhCS.271a2031G. doi:10.1088/1742-6596/271/1/012031. S2CID 4776237.
- ^ The Borexino Collaboration (2020). "Experimental evidence of neutrinos produced in the CNO fusion cycle in the Sun". Nature. 587 (?): 577–582. arXiv:2006.15115. Bibcode:2020Natur.587..577B. doi:10.1038/s41586-020-2934-0. PMID 33239797. S2CID 227174644. Archived from the original on 27 November 2020. Retrieved 26 November 2020.
- ^ Jump up to:
- a b c Phillips, K. J. H. (1995). Guide to the Sun. Cambridge University Press. pp. 47–53. ISBN 978-0-521-39788-9.
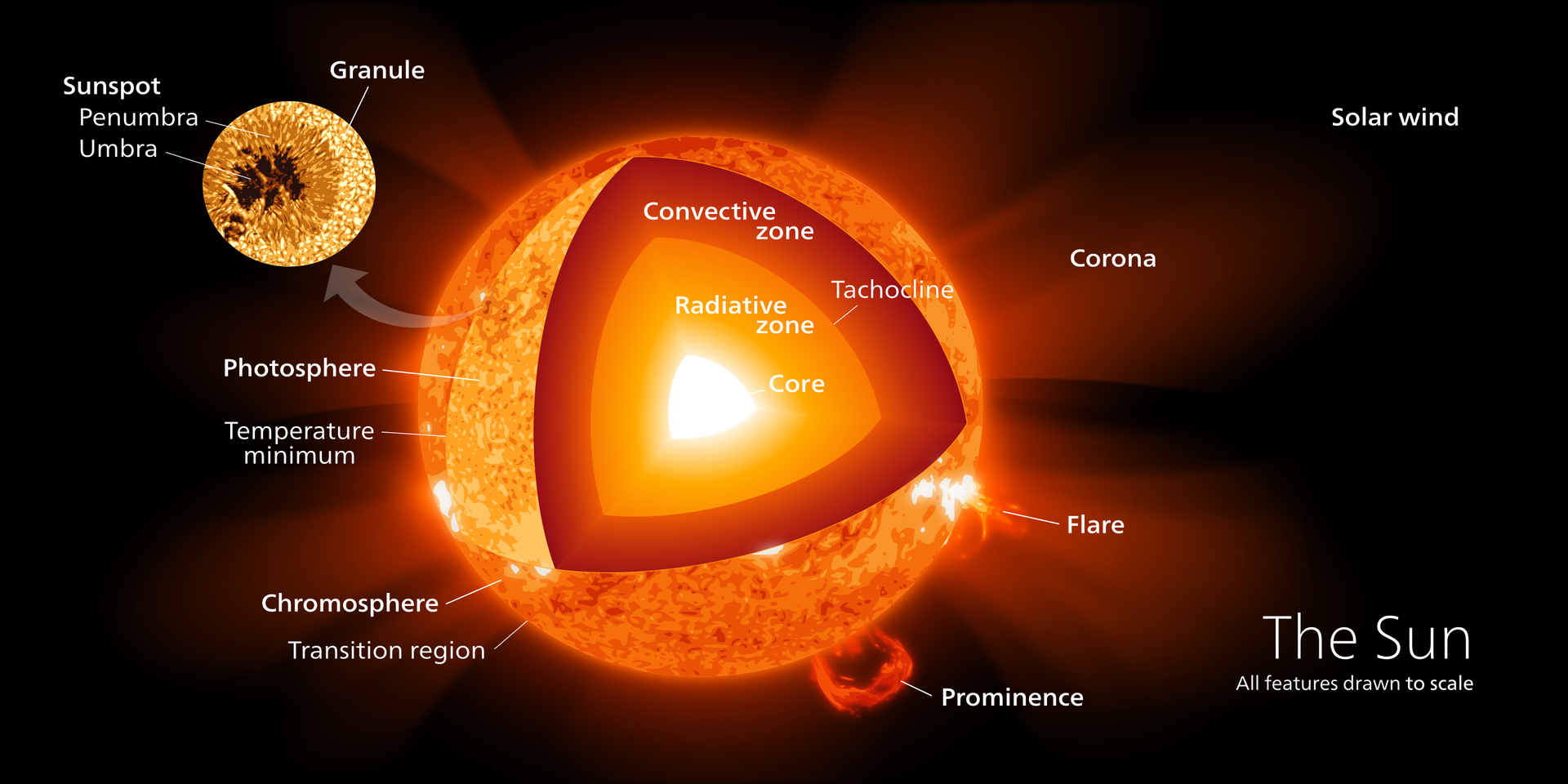
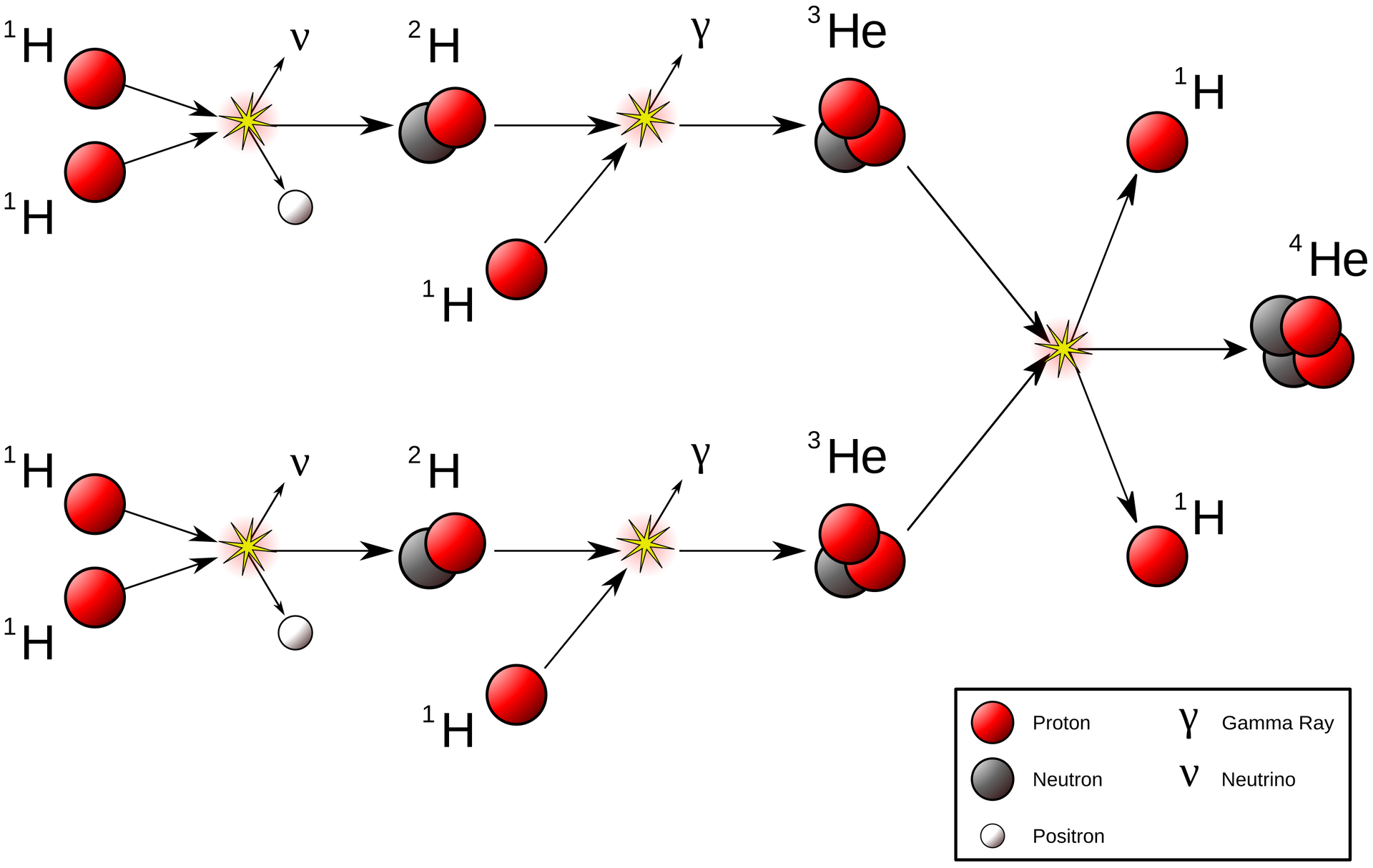
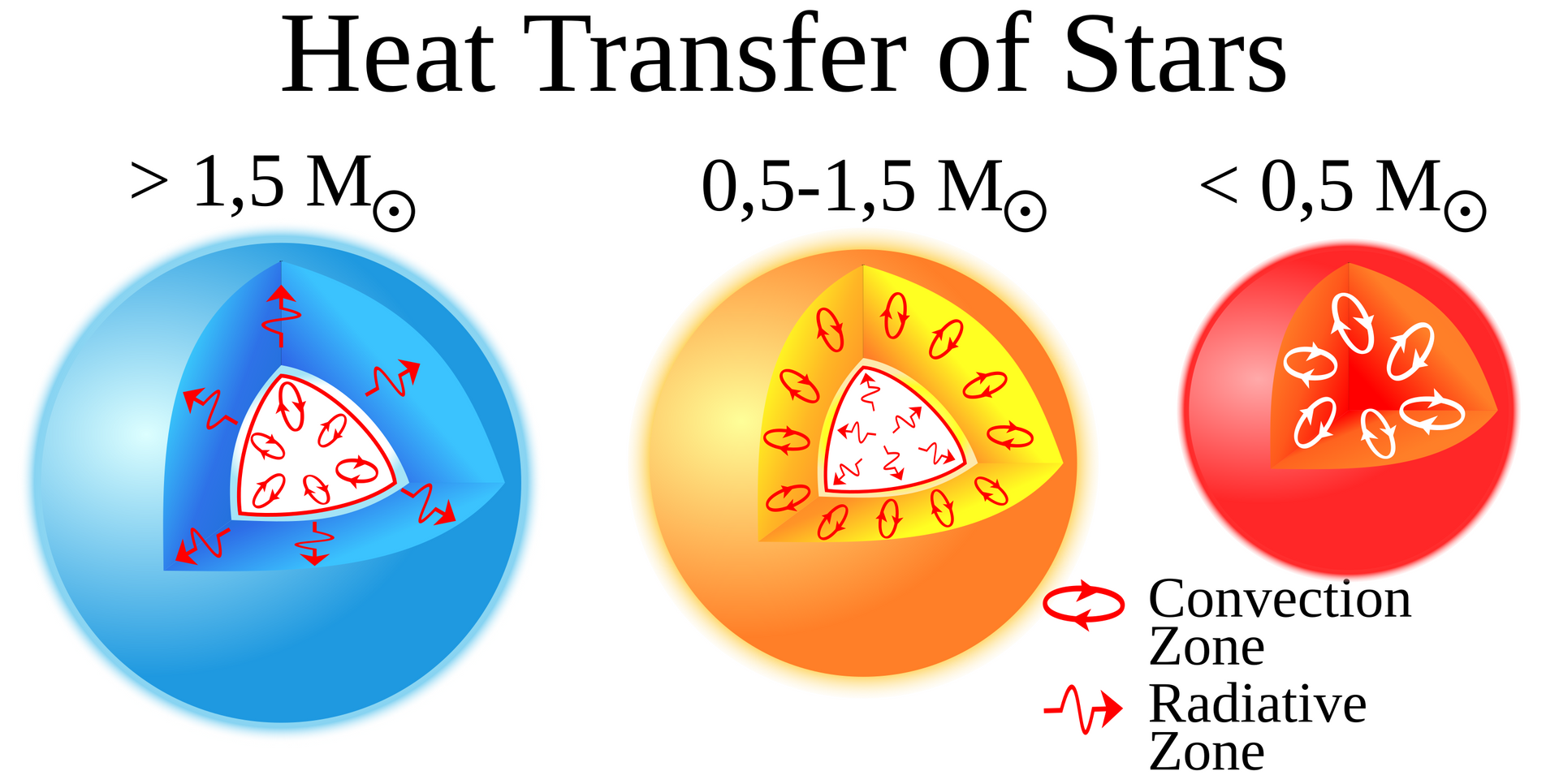